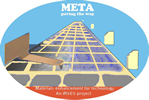
WorkPackage 2 “ Devices based on interface-enhanced conductivity”
(WP coordinator: Carla Andreani)
Objectives
Brief description: The work package aims at nanometer scale investigations of the conductivity in thin films of ion conducting solid oxides. Both oxygen ion and proton conducting materials will be investigated. The final goal is to reach a full understanding of the interplay on a nanometric scale between ionic conductivity and local physico-chemical properties such as electronic conductivity, surface morphology, defects, surface mechanical properties and residual strain. Achieving such objective would be of great importance for fundamental science as well as of technological relevance for the development of micro Solid Oxide Fuel Cells (μSOFCs).
The growing demand for miniaturized systems for energy conversion and storage has prompted our research towards the development of solid state ionic devices in thin film form. Electrochemical devices, such as solid oxide fuel cells (SOFCs), can be miniaturized for portable applications (μ-SOFCs) [2.1]. This goal requires the reduction of the operating temperature and the production of components as thin films [2.2-2.7]. In general, μ-SOFC devices are expected to produce energy densities per volume and specific energy per weight up to four times larger than state-of-the-art rechargeable Li-ion and Ni metal hydride batteries [2.8]. Pulsed Laser Deposition (PLD) has been chosen as the technique for the fabrication of thin solid electrolyte layers because it allows to control composition of the deposited layers at the atomic level.
In detail the group at NAST focuses on the optimization of the synthetic procedures to obtain nanometrically chemically and morphologically tailored electrolytes, i.e. ion conducting oxides or mixed ionic-electronic conductors, and electrode materials, i.e. composite porous structures with extended triple phase boundary.
Electrolyte materials will be deposited by PLD as thin films and heterostructures on different substrates to understand the interplay between crystallographic misfit and electrochemical properties.
The samples will be characterized by Electrochemical Impedance Spectroscopy. The parameters derived from an EIS spectrum fall generally into two categories: (a) those pertinent only to the material itself, such as conductivity, dielectric constant, charge mobility, equilibrium concentrations of the charged species, and bulk generation–recombination rates; and (b) those pertinent to an electrode–material interface [2.9]. EIS allows measuring physical quantities averaged over macroscopic dimensions of the order of the distance between electrical contacts.
Local (on a nanometric scale) information are of paramount importance for a better understanding of the mechanisms linking interface ionic conductivity with other physical and chemical parameters such as local composition fluctuations, epitaxial strain and crystallographic defects.
In this framework a major goal of WP2 is the application of the scanning probe microscopy (SPM) technique developed by the ORNL partners to map the local ionic conductivity. The SPM technique allows investigating ionic conductivity in very thin layers where the spurious contribution from the substrate hinders the utilization of standard EIS techniques.
The ORNL-CNMS group has been actively involved in the development of local SPM methods for probing electrochemical phenomena and frequency dependent transport on the nanoscale. These include Nanoimpedance Microscopy [2.10], which combines the AFM probe and classical impedance spectroscopy to gain spatially-resolved impedance data. The team at ORNL has demonstrated the feasibility of mapping ionic transport on the nanoscale through the detection of bias-induced strains. These unique sets of experimental techniques will be used to address local transport in solid ionic and ionic-electronic conductors in different geometries.
In addition to the mapping results available from scanning probe microscopy, neutron spectroscopy will be applied to provide important information on the dynamics of ions in SOFC materials These investigations will enable a more complete description of ionic and electronic conductivity over a broad range of length and time scales.
Neutron spectrometers at the Spallation Neutron Source (SNS) at ORNL are used to provide this critical information on ionic conductors. In the temperature regime where the ions are mobile, quasi-elastic scattering measured on NSE, BASIS, and CNCS instruments allows to understand the different time scales of the dynamics as influenced by the lattice. Additional information on the potentials that the affect the ions can be extracted by measuring the inelastic data just above and just below the temperature where the ions become mobile. Of particular interest are the inelastic modes that remain once the ions become mobile. These data would be acquired on ARCS or SEQUOIA and would be compared directly to computational predictions of the density of states.
Deep Inelastic Neutron Scattering (DINS) will also be used to determine the proton momentum distribution function, n(p) which complements the collective response probed by neutron scattering at relatively low energy techniques. This distribution, which is determined almost entirely by quantum effects for the systems studied, is a sensitive probe of the local environment of the proton, as well as a direct measurement of its dynamics [2.11].
The final goal of this work package is to reach a satisfactory understanding of the interplay between ionic conductivity and interface properties.
List of References:
[2.1] U. P. Muecke, D. Beckel, A. Bernard, A. Bieberle-Hütter, S. Graf, A. Infortuna, P. Müller, J.L.M. Rupp, J. Schneider, L.J. Gauckler; Micro Solid Oxide Fuel Cells on Glass Ceramic Substrates. Adv. Funct. Mater. 2008, 18, 3158-3168
[2.2] A. Bieberle-Hütter, J.L. Hertz, H.L. Tuller; Fabrication and electrochemical characterization of planar Pt-CGO microstructures. Acta Mater. 2008, 56, 177-187
[2.3] J. Fleig, H. L. Tuller, J. Maier; Electrodes and electrolytes in micro-SOFCs: a discussion of geometrical constraints. Solid State Ionics 2004, 174, 261-270
[2.4] J. H. Joo, G. M. Choi; Electrical conductivity of thin film ceria grown by pulsed laser deposition. J. Eur Ceram Soc. 2007, 27, 4273-4277.
[2.5] J. L. Hertz, H. L. Tuller; Electrochemical characterization of thin films for a micro-solid oxide fuel cell. J. Electroceram. 2004, 13, 663-668
[2.6] N. Ito, M. Iijima, K. Kimura, S. Iguchi; New intermediate temperature fuel cell with ultra-thin proton conductor electrolyte . J. Power Sources 2005, 152, 200-203
[2.7] S. J. Litzelman, J. L. Hertz, W. Jung, H. L. Tuller; Opportunities and Challenges in Materials Development for Thin Film Solid Oxide Fuel Cells. Fuel Cells 2008, 5, 294-302
[2.8] A. Bieberle-Hütter, D. Beckel, A. Infortuna, U.P. Muecke, J.L.M. Rupp, L.J. Gauckler, S. Rey-Mermet, P. Muralt, N.R. Bieri, N. Hotz, M.J. Stutz, D. Poulikakos, P. Heeb, P. Müller, A. Bernard, R. Gmür, T. Hocker; A micro-solid oxide fuel cell system as battery replacement. J. Power Sources 2008, 177, 123-130
[2.9] E. Barsoukov, J. Ross Macdonald; Impedance Spectroscopy Theory, Experiment, and Applications. John Wiley & Sons, Inc., Publication.
[2.10] R. Shao, S. V. Kalinin, D. A. Bonnell; Local impedance imaging and spectroscopy of polycrystalline ZnO using contact atomic force microscopy. App. Phys. Lett. 2003, 82, 1869-1871
[2.11] C. Andreani, D. Colognesi, J. Mayers, G. F. Reiter, R. Senesi; Measurement of momentum distribution of light atoms and molecules in condensed matter systems using inelastic neutron scattering. Advances in Physics 2005, 54, 377-469